KEY TO
GREEN
TRANSITION
Get in touch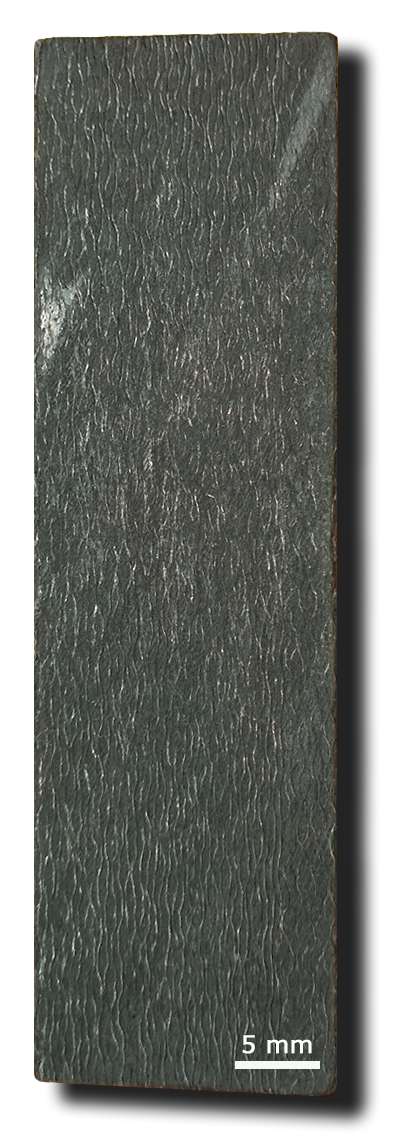
Cross section of carbon fiber composite sample nano-structured by our product for Ultra Lightweighting.
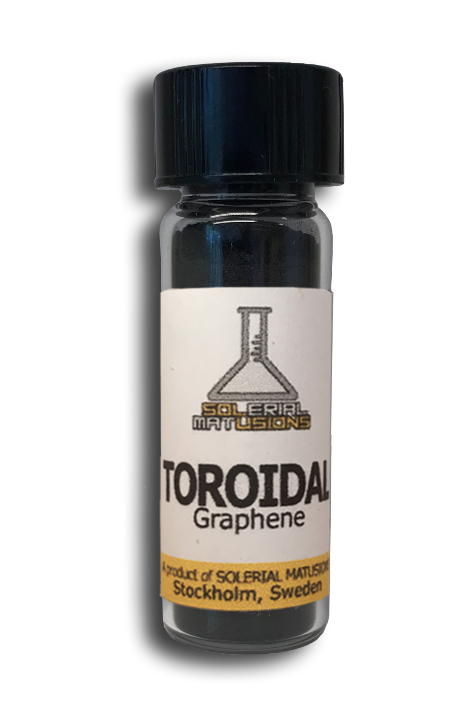
TOROIDAL
GRAPHENE
Key to electrification
The unique selling point of our patented product, Toroidal graphene, is the capacity to boost the electrical conductivity of copper to quantifiable improvements of 300-375%, a level that exceeds industry demands and significantly outperforms our main competitor, 2D graphene, and translates into tangible benefits such as increased energy efficiency and reduced carbon emissions, which aligns with sustainability goals and supports the green transition.
ELECTRIFICATION
In the quest for a sustainable and low-carbon future, electrification emerges as a crucial catalyst. Its fundamental role lies in facilitating the widespread use of renewable energy and reducing our reliance on fossil fuels. At the core of electrification's importance is the extensive adoption of electric vehicles (EVs), which serve as a key driver. EVs are not just components, but rather integral elements that contribute significantly to the electrification process. By embracing EVs, we propel the agenda of electrification, leading to a reduction in carbon emissions, the integration of renewable energy sources, improved air quality, enhanced energy efficiency, technological innovation, and ultimately, a transition towards a more environmentally friendly future.
Problem
-
- Limited Driving Range: One of the primary barriers to the widespread adoption of EVs is the limited driving range offered by current battery technology. Many consumers are concerned about running out of charge before reaching their destination, leading to range anxiety and hesitation to switch from internal combustion engine vehicles (ICEVs) to EVs.
- Slow Charging Speeds: Another challenge is the relatively slow charging speeds of EVs compared to refueling times for ICEVs. Long charging times can inconvenience drivers, especially during long trips or when charging infrastructure is limited, further exacerbating range anxiety and hindering the adoption of EVs.
Solution
Utilizing conductivity-enhanced copper in the various components of the EV battery system and the charging infrastructure can significantly benefit driving range and faster charging times:
-
- Busbars & interconnects: Conductivity-enhanced copper busbars and interconnects reduce electrical resistance, enabling more efficient flow of electricity between battery cells. This minimizes energy loss during charging and discharging, maximizing the amount of energy available for driving and extending the vehicle’s range. Additionally, the transfer of electricity from busbars to battery cells becomes more efficient, ensuring even distribution of the electrical load across the battery pack. Enhanced conductivity also facilitates faster charging rates, allowing for quicker replenishment of energy during charging sessions.
- Terminals & wiring: Enhanced conductivity in copper terminals and wiring ensures efficient transmission of electricity, reducing energy loss and voltage drops along the wiring. This maximizes the amount of electricity delivered to the battery cells, leading to faster charging times and extended driving range per charge. Faster and more reliable transfer of electricity between the battery pack and the vehicle’s electrical system also results in shorter charging times and quicker acceleration, enhancing the driving range and overall performance of the electric vehicle.
- Cooling plates: Enhanced conductivity in copper cooling plates improves their ability to dissipate heat generated during charging and discharging cycles. Efficient heat dissipation helps maintain optimal operating temperatures within the battery pack, preserving battery life and ensuring consistent performance. This allows for faster charging without compromising safety or longevity.
In the charging infrastructure, conductivity-enhanced copper reduces electrical resistance in cables and connectors, minimizing energy loss during the charging process. This means that more of the electricity delivered from the charging station reaches the vehicle’s battery, maximizing the amount of energy available for driving and extending the vehicle’s range per charge. Additionally, lower resistance enables faster transfer of electricity from the charging station to the vehicle’s battery, resulting in shorter charging times and enhanced practicality of EVs. Conductivity-enhanced copper components in the charging infrastructure also contribute to optimized distribution of electricity, compatibility with high-power charging, and enhanced thermal management, further improving charging times, driving range, and overall usability of electric vehicles.
By addressing key barriers such as limited driving range and slow charging speeds, the introduction of Toroidal graphene can accelerate the widespread adoption of EVs. Increased driving range, faster charging times, and improved affordability make EVs more appealing to consumers, driving market growth and promoting a transition to sustainable transportation. As the sole provider of Toroidal graphene, we offer unparalleled advantages and opportunities for investors and stakeholders seeking to capitalize on the rapid growth of the EV market and beyond.
INDUSTRY TRACK RECORD
The recent collaboration between Solerial Matusions, Scania, and the Swedish Metals Research Institute (Swerim) in a government-funded pre-study to develop a lead-free Toroidal graphene-Bronze alloy for truck gearbox pads showcases an impressive industry track record in several key areas:
-
- Innovative Material Development: Solerial Matusions’ successful participation in this collaborative project demonstrates its capability to innovate and develop advanced materials tailored to specific industry needs. The creation of a lead-free Toroidal graphene-Bronze alloy with significantly higher wear resistance than traditional leaded bronze highlights the company’s commitment to pushing the boundaries of materials science.
- Technical Expertise and Research Collaboration: By partnering with Scania and Swerim, Solerial Matusions has demonstrated its ability to collaborate with industry leaders and research institutions to tackle complex challenges. This collaboration underscores the company’s technical expertise and its ability to leverage external resources to achieve breakthrough results.
- Superior Performance and Technological Advancement: The tribological evaluation showing a 324% increase in wear resistance compared to leaded bronze signifies a significant technological advancement. The ability of the new alloy to dissipate frictional heat more efficiently, leading to lower operating temperatures and reduced thermal-induced wear, demonstrates Solerial Matusions’ ability to deliver materials with superior performance characteristics.
- Market Potential and Customer Endorsement: Scania’s interest in Solerial Matusions’ new alloy positions the truck manufacturer as a potential customer, indicating market potential and commercial viability. This endorsement underscores the relevance and value of Solerial Matusions’ technology in addressing real-world industry needs.
- Strategic Planning for Commercialization: The acknowledgment of the need for funding to scale production indicates strategic planning and foresight. Solerial Matusions’ recognition of the importance of securing resources for scaling demonstrates a proactive approach to commercialization and growth within the industry.
Overall, this latest collaborative project exemplifies Solerial Matusions’ industry track record by showcasing its ability to innovate, collaborate, and deliver high-performance solutions that address specific industry challenges. It highlights the company’s technical expertise, commitment to advancement, and potential to capture market opportunities in the automotive sector.
INDUSTRIAL
CASE STUDIES
The performance enhancement capability of Toroidal graphene has been documented by us in several industrial case studies, where components made of composite materials nano-structured by Toroidal graphene at concentrations of 0.0025-0.01wt% was demonstrated under real operational conditions (TRL-level 7):
• The collaboration between Solerial Matusions, Scania, and the Swedish Metals Research Institute (Swerim) in 2022 in a pre-study to develop a lead-free bronze alloy for truck gearbox pads resulted in the creation of a lead-free Toroidal graphene-Bronze alloy with significantly higher wear resistance than traditional leaded bronze. The tribological evaluation showed a 324% increase in wear resistance compared to leaded bronze as well as the capability to dissipate frictional heat more efficiently, leading to lower operating temperatures and reduced thermal-induced wear.
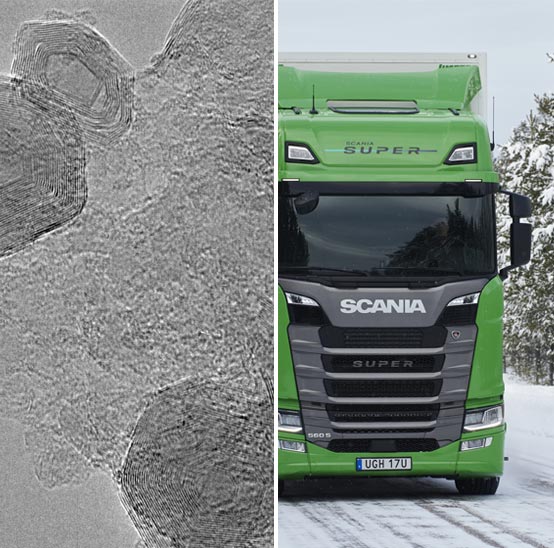
• A grinding support made of nano-structured bronze (tin copper) was tested by Thyssen in Germany. The test showed an improvement in abrasive wear resistance of 500% compared to Thyssen’s grinding supports. Hardness and electrical conductivity improvements were measured to 40% and 375%, respectively. The size of the improvement in electrical conductivity by adding such ultra-low concentrations of our product proves that the toroidal graphene molecule has an intrinsic electrical conductivity of at least 600-750 times higher than copper.
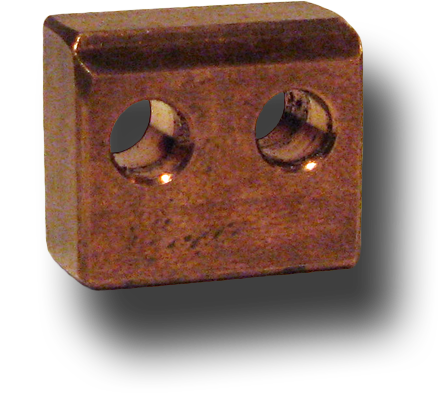
• In an experimental study to find a substitute for the toxic beryllium copper, beryllium was replaced by our product. Mechanical properties were preserved, and electrical conductivity improved by 300%.
•A tool holder for turning was made of nano-structured carbon fiber composite. In demonstrations it allowed turning at 200,000 rpm with a maximum tool deflection of 2 mm, which is an improvement by a factor of 20 compared to steel. SP Technical Research Institute of Sweden conducted independent testing of the compression modulus of the tool holder, which verified a performance enhancement of over 40% compared to Japanese world-leading carbon fiber manufacturer Toray.
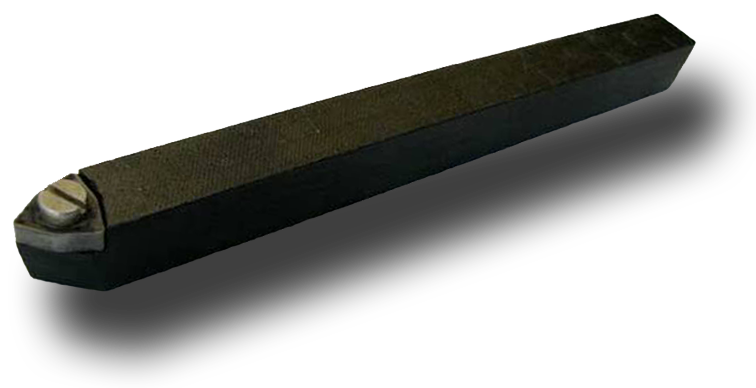
• A pervaporation membrane for separating azeotropic mixtures made of nano-structured thermoplastic (PPO) didn’t influence the pervaporation properties but showed an improvement in biaxial tensile strength of 85%.
• Chemical resistance to nuclear waste in borosilicate glass, nano-structured with our product, has been measured to 400% improvement.
• Laboratory environment feasibility studies have shown improvements in tensile strength of epoxy by 39%; in flexural modulus of T700 carbon fiber fabric by 45%, which is equivalent to T1300-level; and in flexural modulus of engineering plastics by 54%.
TECHNOLOGY
Toroidal graphene molecule
The toroidal graphene molecule is a nano-scientifically engineered zero-dimensional1 sp2-hybridized carbon allotrope, consisting of 20-40 defect-free graphene layers without function-group content, geometrically fixed in a closed conjugated system with delocalized pi-electrons interacting freely in circular motion in the closed loop of the cyclic structure of the toroid instead of being occupied in pi-bonds with adjacent graphene layers. Thus, the toroidal graphene molecule exhibits delocalization of pi-electrons from the multiple layers in coherent oscillations across the entire molecule.
1. 0D nanomaterials have zero dimensions larger than the nanoscale and thus have all three dimensions confined at the nanoscale, as opposed to 2D nanomaterials like 2D graphene, which have two dimensions larger than the nanoscale, and one dimension confined at the nanoscale. Nanoscale refers to dimensions on the order of nanometers, ranging from 1 to 100 nanometers.
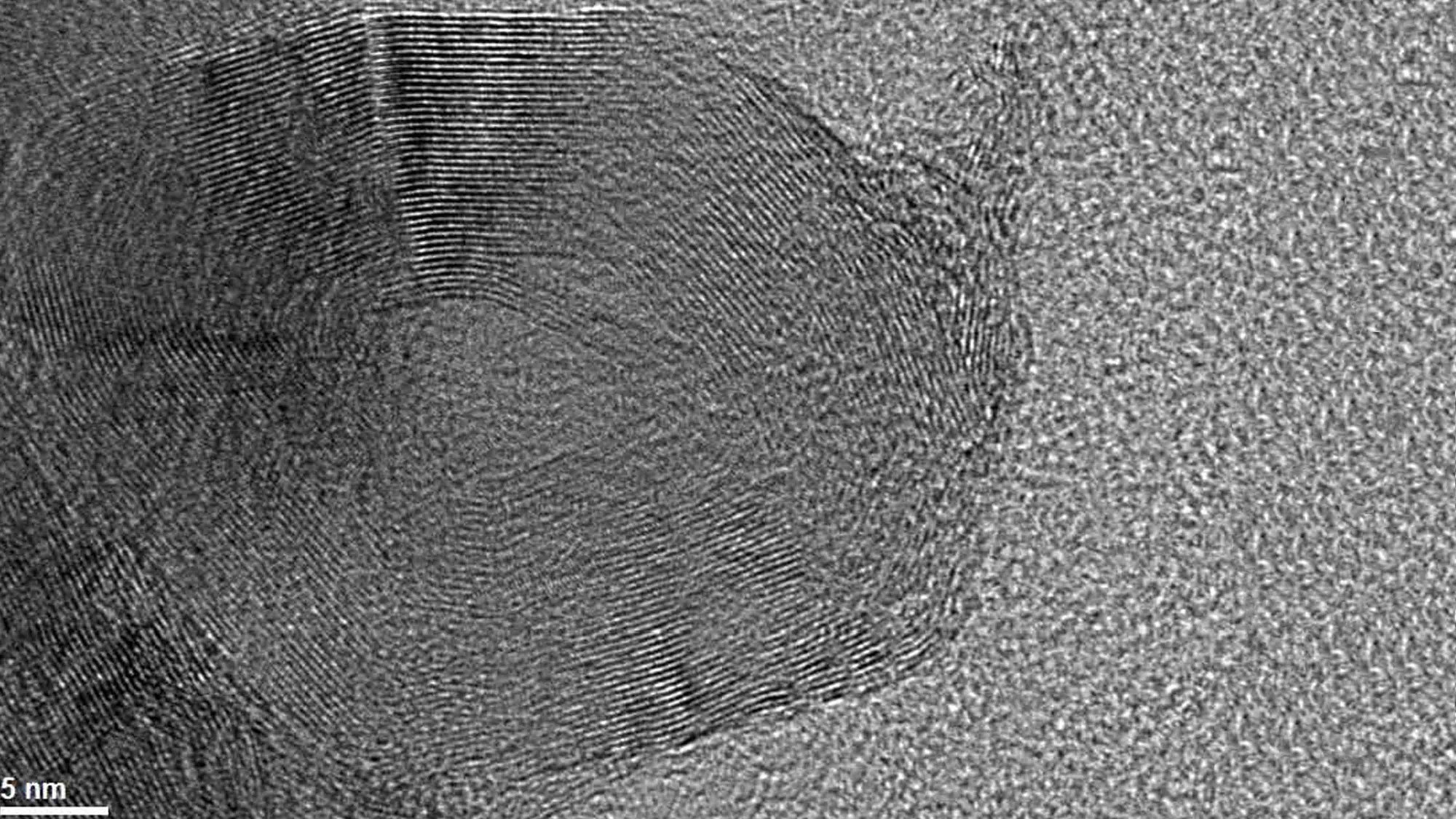
Giant resonance
The coherent motion of delocalized pi-electrons arises from quantum mechanical principles, where neighboring pi-electrons exhibit wave-like constructive interference patterns, which occur when the wavefunctions of pi-electrons of the multiple layers align in phase, leading to an amplification of the oscillations that ultimately trigger a resonant response.
This resonance phenomenon, known as giant resonance, leads to a significant enhancement of the electromagnetic field of the molecule, amplifying its intensity by a factor of 30,000, as observed in Raman scattering experiments, where the amplification results in stronger Raman signals, providing direct evidence of the giant resonance phenomenon.
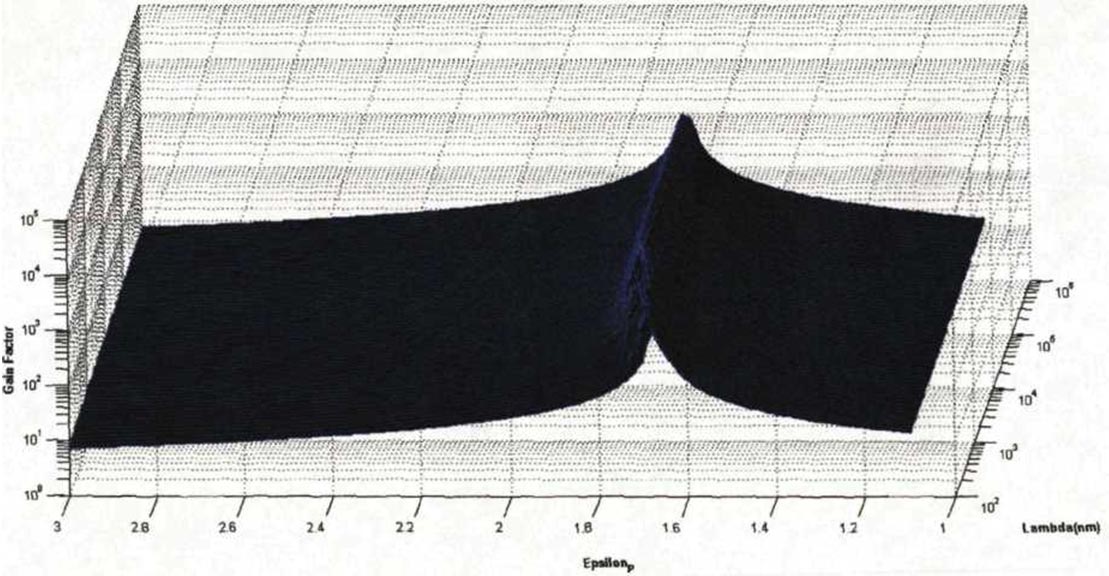
NANO-
STRUCTURING
CAPABILIITY
Extreme electron mobility together with exceptional structural and thermal integrity makes Toroidal graphene the ideal nanostructuring agent for enhancing composite materials such as metal alloys, engineering plastics, and carbon fiber.
The incorporation of toroidal graphene molecules into a composite material significantly influences its overall performance. When uniformly dispersed, they establish a stable internal network within the material. The network stability comes from the alignment and attraction of temporary dipoles between the toroidal graphene molecules, a phenomenon known as van der Waals forces.
The van der Waals forces exerted by the toroidal graphene molecules demonstrate heightened strength and expanded range, owing to modified electron density and electronic polarization induced by giant resonance.
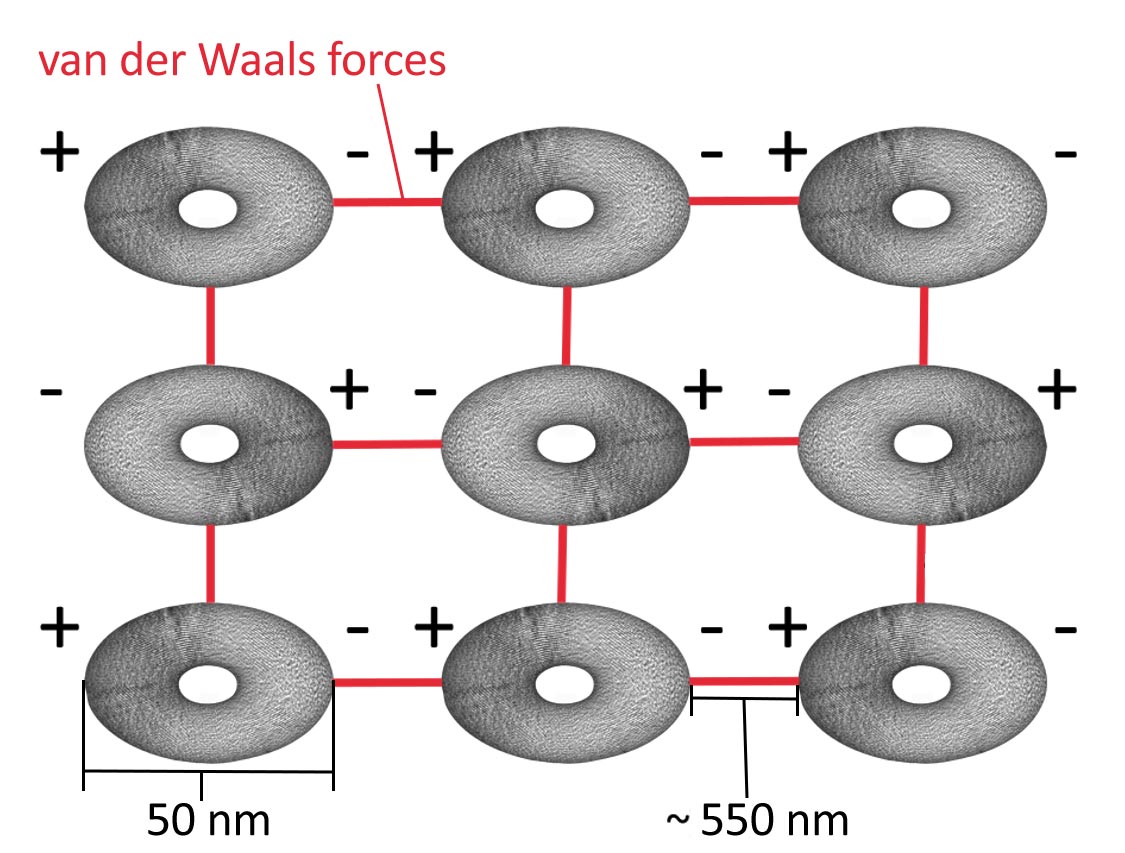
The interlayer shear properties of the toroidal graphene molecule are characterized by exceptionally high shear strength, preventing the individual graphene layers from being separated, even during extreme processing conditions. This phenomenon arises from the strong covalent bonding within each defect-free graphene layer and the quantum mechanical interlayer interactions, which collectively resist shear deformation and ensure structural integrity.
Densified interfacial layers
Dispersing toroidal graphene molecules uniformly in a copper matrix with a spacing of 550 nm, and thereby ensuring optimal interaction between the temporary dipoles of adjacent toroidal graphene molecules, promotes the formation of denser, more compact interfacial layers between the toroidal graphene molecules and the surrounding copper atoms.
Amplified van der Waals forces between the toroidal graphene molecules and the copper atoms play a crucial role in promoting stronger interactions at the interfaces. The attractive forces pull the copper atoms closer to the toroidal graphene molecules, thereby contributing to the densification of the interfacial layers with improved cohesion and adhesion.
Superior electrical and thermal conductivity
The densified interfacial layers within the nanostructured copper facilitate better contact between the toroidal graphene molecules and the copper atoms, improving transfer pathways and mobility of charge carriers and phonons through the composite material, as well as reducing scattering, and minimizing energy losses, ultimately reducing resistance and thus contributing to higher electrical and thermal conductivity.
Stronger intermolecular interactions also lead to a smoother surface and reduced frictional resistance, resulting in improved durability and longevity of the material.
In the case of carbon fiber composites, an interfacial layer is formed around the carbon fibers as well.
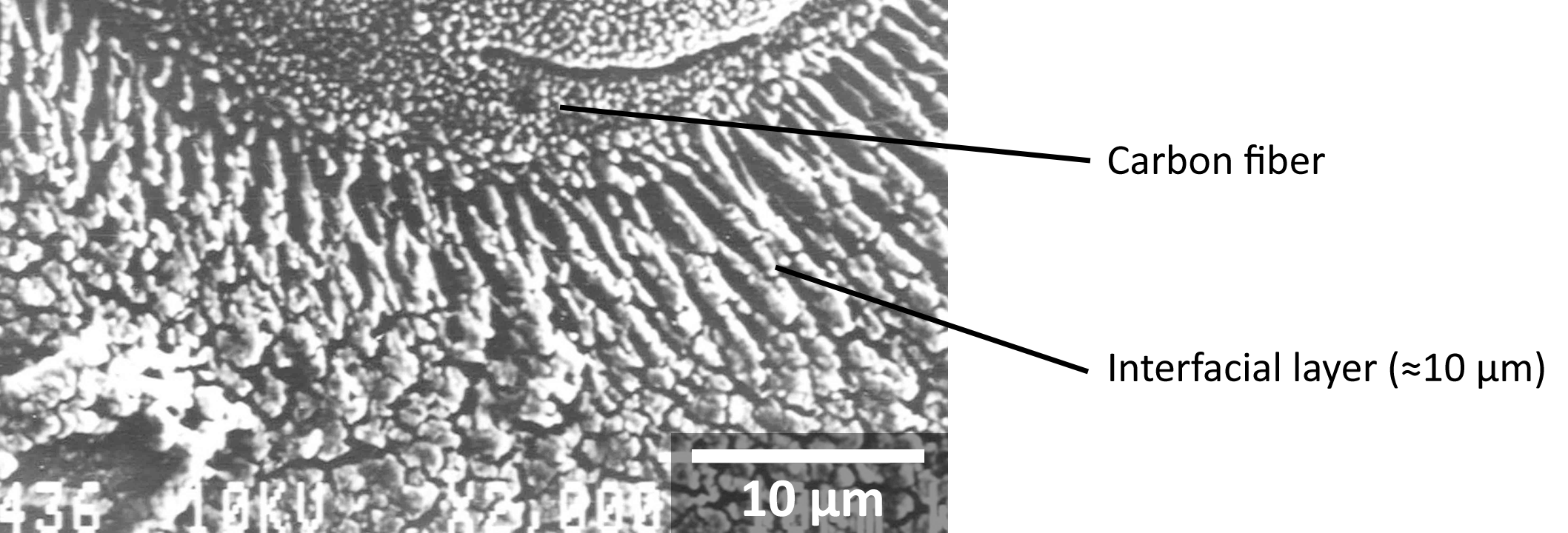
COMPETITION
Competing technologies in the nanomaterial industry are mainly based on Carbon nanotubes (CNTs) or 2D graphene.
Carbon nanotubes
CNTs have been used as an additive in tens of thousands of attempts to modify materials and exits from this market include Bayer AG which described the potential applications of CNTs as ‘fragmented with comprehensive commercialization not likely in the foreseeable future’.
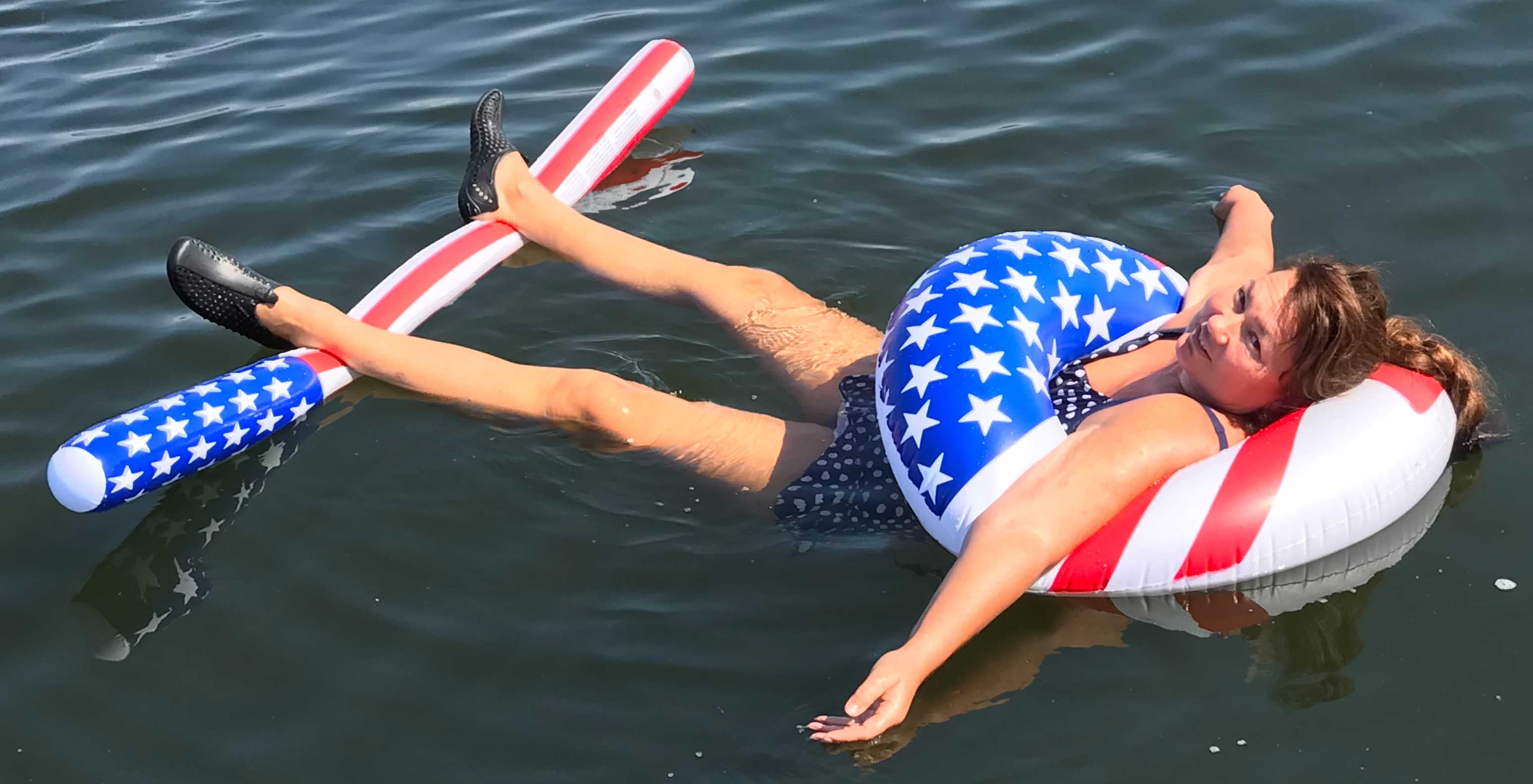
2D graphene
In the competitive landscape of nanomaterials, 2D graphene stands out as a key player, having been recognized by the Nobel Prize in Physics in 2010 for its remarkable properties. However, while the nanostructure of the graphene layer holds great promise, realizing its potential in real-world applications requires exploring alternative graphene solutions that offer defect-free graphene layers with greater structural and thermal integrity, such as the toroidal graphene molecule.
AEROSPACE
ULTRA
LIGHT-
WEIGHTING
With a systems-level approach, which involves considering the potential for ultra lightweighting from the beginning of the design process, it is possible to optimize the design of a 300-seat, 140-ton carbon fiber jet with a list price of $325m.
One kilogram of our product ultra-lightweights the carbon fiber parts of a 140-ton carbon fiber jet and addresses the following five primary areas of aerospace lightweighting:
• Weight savings – Our product allows for weight savings of 40% of the carbon fiber composite parts or a total of 28 tons, which equals cost savings of $8.7m.
• Fuel consumption – Our product allows for a reduction in fuel consumption by 20% or 10+ tons of fuel for a single Atlantic crossing, which equals cost savings of $10m over the life of the aircraft.
• CO2 emissions – Our product allows for a reduction in CO2 emissions by 20% or 30+ tons for a single Atlantic crossing.
• Range – Our product improves range flexibility by 20% or 2,800 km, thereby increasing the number of possible non-stop destinations around the world.
• Aircraft lightning protection – Carbon fiber composites cannot mitigate the potentially damaging electromagnetic effects from a lightning strike. The most frequently selected solution to this problem is to introduce metals back into the aircraft. The metal mesh and expanded foil added to the composite structure layup for this purpose are negating any lightweighting measures and don’t contribute to structural strength. Carbon fiber composites enhanced with our product serves as an electrical shield around the structure that protects against lightning strikes and at the same time contributes to structural strength.
OPPORTUNITY:
WORLD
LEADING
CARBON
FIBER
COMPOSITE
Any carbon fiber manufacturer with T800-level carbon fiber could with the help of our product surpass the current world leader in carbon fiber composites, Toray of Japan, by more than 30% or nearly 1,000 MPa, which will be the biggest leap in the evolution of carbon fiber composites in over 30 years.
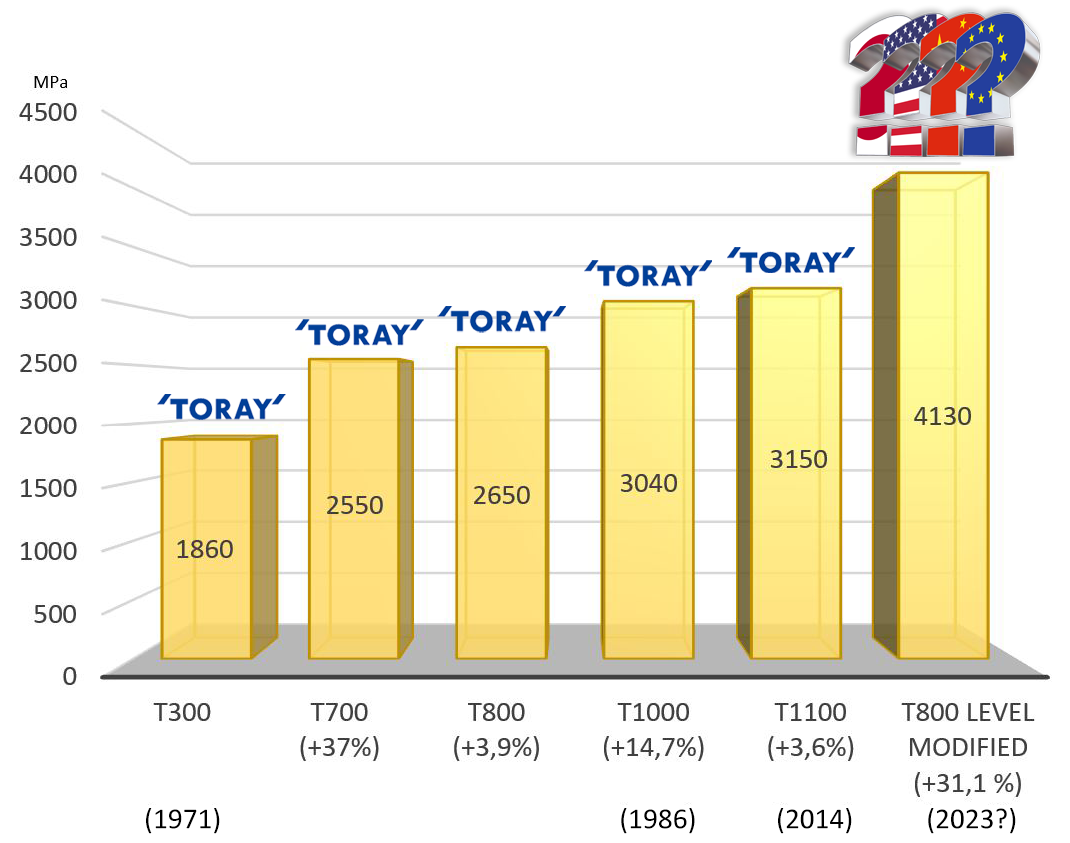
SUSTAINABILITY
Our product’s capability to enhance the electrical conductivity of copper aligns with multiple United Nations' Sustainable Development Goals (SDGs) for 2030, particularly in the areas of industry, innovation, and infrastructure; clean energy; and responsible consumption and production.
- Affordable and Clean Energy (SDG 7):
- Improved electrical conductivity can enhance the efficiency of electrical systems, reducing energy loss during transmission and distribution.
- Higher conductivity allows for more efficient energy generation, contributing to the goal of affordable and clean energy.
- Industry, Innovation, and Infrastructure (SDG 9):
- Enhanced electrical conductivity can lead to the development of more efficient and innovative electrical infrastructure and technologies.
- Improved conductivity can contribute to the advancement of electric vehicles, renewable energy systems, and smart grids, supporting sustainable industrialization and infrastructure development.
- Responsible Consumption and Production (SDG 12):
- By increasing the efficiency of electrical systems, the product can contribute to reduced resource consumption and waste associated with energy production and transmission.
- The enhanced conductivity may lead to longer-lasting and more reliable electrical components, promoting sustainable consumption patterns.
- Climate Action (SDG 13):
- Increased electrical conductivity can contribute to the development and adoption of cleaner energy technologies, thereby mitigating the impact of climate change.
- Efficient energy transmission and reduced energy loss can result in lower greenhouse gas emissions associated with energy production and consumption.
- Partnerships for the Goals (SDG 17):
- Collaborations between the manufacturers of the product, the copper industry, and other stakeholders can foster partnerships aimed at achieving sustainable development goals.
- Sharing knowledge and technologies related to enhanced electrical conductivity can contribute to a collective effort to address global challenges.
LET'S GET
TOGETHER.
If you believe that we may have a technology that could be of benefit to your company ...
or if you just want to chat …
Project partners